Controlling chemical reactions and their outcomes has been a chemist’s dream for ages. Understanding chemical interactions, internal energy transfers, and reactivity to external inductions are necessary for taming the molecules. Nevertheless, the Nature is trying to trip us chemists there is light in the darkness of knowledge.
Teksti Jan Lundell
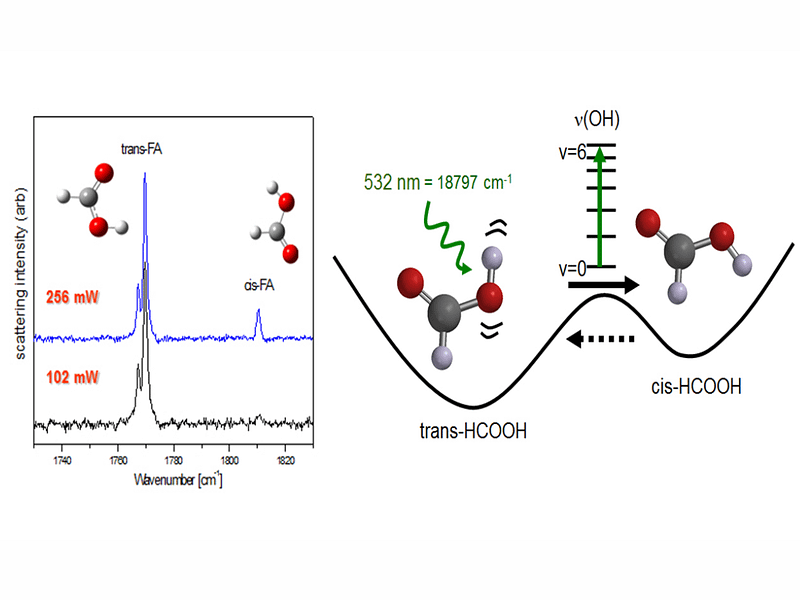
Chemistry is very complicated even though we have equipment and skills as demonstrated by the Nobel prize in chemistry given for AI-modelling of proteins. To unravel the inner life of molecules experimentally we still need model systems to start with.
We also need to simplify the multimodal environments of life for simple but relevant environments that allow systematic, accessible and reliable scientific approaches.
Low temperature matrix isolation technique was originally developed in the 1950’s to trap chemical entities in solid, non-reactive and isolating surroundings. Especially the noble gases in the temperature range of 4–50 K have turned to be beneficial as isolating media.
The approach has been used to isolate reactive species like the OH radical, to study the outcome of laser-ablated metal clusters, tracking the photodecomposition pathways of carboxylic acid molecules, and characterizing novel chemical species like the first neutral argon-containing molecule HArF discovered at Helsinki University in 2000.
The advent of tunable light sources presents another possibility to study molecules on surgical precision.
Choosing a suitable wavelength of radiation, the molecules can be tickled in IR, UV or visible light regions to induce vibrational or electronic structure changes.
For isolated chemical systems in low temperatures, this allows us to induce, monitor and understand the interplay of matter and light for the benefit to understand how chemical reactions proceed and what controls the outcome of a chemical reaction.
Selective light for selective chemistry
Selective chemistry in low temperatures was first thoroughly demonstrated for studies on formic acid, HCOOH. This was achieved by IR-pumping energy into the first vibrational overtone of OH stretching more.
Thereafter, it has been shown that almost any vibrational fundamental or overtone vibration can be used as a power intake channel in molecules. For formic acid this was employed for C-H and O-H stretching fundamental modes along with several vibrational overtone vibrations.
The consequence of selective IR-pumping was always that the higher energy form cis HCOOH was generated and identified. Even in low temperatures the new species was observed to tunnel back to the most stable form in a matter of minutes. In ambient conditions on Earth only the lowest-energy form trans-HCOOH exists, even though the higher-energy conformer has been detected in space, especially in the great molecular cloud Sagittarius A in the Sagittarius constellation.
Selectivity for purpose
The opportunity to produce selectively higher-energy molecules is intriguing. Under UV-photolysis formic acid decomposes to H2+CO2 and H2O+CO. The ratio of these is 50/50 for trans-HCOOH, but 90/10 for cis-HCOOH.
This demonstrates a “conformational memory” effect upon photolysis. The photolyzed, original molecular structure affects the formation of photoproducts and their abundance.
Formic acid has turned out to be a perfect guinea pig for selective light-molecule interaction studies. The findings of IR-photolysis of monomeric molecule can be extended to molecular complexes. For example, for formic acid dimer many trans-trans, trans-cis and cis molecular complex structures have been created and identified.
Tuning the light into visible light the same chemical processes can be ignited but employing different energy intake channels. Such studies can be used to study the efficiency of light-induced processes on various conformer structures of the same molecule for the benefit for most advantageous chemistry to be achieved.
From laboratory to real life
Recently, we have together with prof. Theo Kurten at Helsinki University studied the possibility of using tunable light sources for selective chemistry to understand molecular mechanisms in atmospheric chemistry.
The target was to employ the light-low-temperature combination experiments to study the possibility to produce reactive intermediate radicals suggested to appear during atmospheric chemical reactions. This has turned out to be very tricky to even for model systems because of subsequent chemical reactions or very short lifetimes of the chemical species.
On the other hand, molecular interactions mediated by hydrogen bonds have shown an interesting aspect related to atmospheric and astrochemistry.
Weak chemical interactions deform the complex subunit structures. This leads to changes in their interaction susceptibility with photons of certain wavelengths. When molecular structure changes also the vibrational modes shift due to the changes.
Shortly, molecular complexes provide new energy intake channels for energy not only via shifter vibrational modes but also through the vibrational modes connected to intermolecular chemical interactions. This can have significant effect on our understanding how atmospheric chemistry is affected by light.
We might also ask how the added thermal energy flows adding up for the warming atmosphere of earth. Consequently, chemistry research in low temperature in laboratory can provide explanations on chemistry on large-scale phenomena of matter of life.
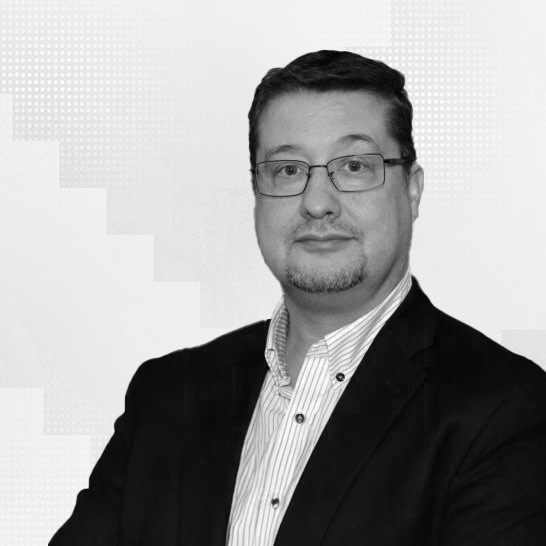
Jan Lundell
Chemistry professor at the University of Jyväskylä. His research interests are in physical and computational chemistry, especially in interactions between light and matter, as well as weak chemical interactions like hydrogen bonding and other non-covalent interactions.
He also has a long experience in chemistry education and chemistry teacher training.
He is the PI of recent Academy of Finland research projects Vibrational excitation induced chemistry (2015–2019) and Light-induced controlled chemistry of atmospheric complexes (2021–2024).
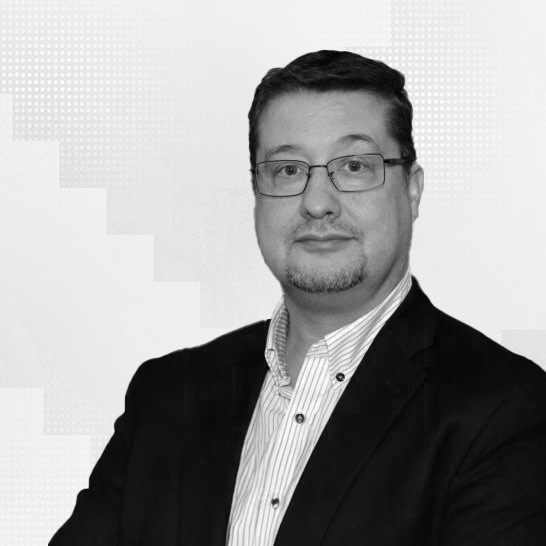
Jan Lundell
Chemistry professor at the University of Jyväskylä. His research interests are in physical and computational chemistry, especially in interactions between light and matter, as well as weak chemical interactions like hydrogen bonding and other non-covalent interactions.
He also has a long experience in chemistry education and chemistry teacher training.
He is the PI of recent Academy of Finland research projects Vibrational excitation induced chemistry (2015–2019) and Light-induced controlled chemistry of atmospheric complexes (2021–2024).
Tilaa uutiskirjeemme!
Saat uusimmat uutiset suoraan sähköpostiisi
95 %
On tyytyväisiä
500
Uutta työntekijää
2000
lukijaa